On a fundamental level, computing systems rely on the ability to store and manipulate information represented and stored as a stream of electrical or optical pulses in the form of binary states 0 and 1. On the other hand, Quantum computers leverage quantum mechanical phenomena to manipulate information. To do this, they rely on quantum bits or qubits, which are typically subatomic particles such as electrons or photons. Companies use superconducting circuits cooled to temperatures colder than deep space to isolate the qubits in a controlled quantum state. The two-level system of a qubit exhibits quantum mechanics properties like ‘superposition’, and ‘entanglement’. An atom’s electrons decay and stay intact at the same time, and in the same way, Qubits can represent numerous possible combinations of 1 and 0 at the same time. This ability of the qubits to be simultaneous in multiple states is called superposition. They are much like the zeroes and ones of the present binary system, the difference being that usually understood zero or one is either a zero or a one or both at the same time and the information exists in either state at the same time.
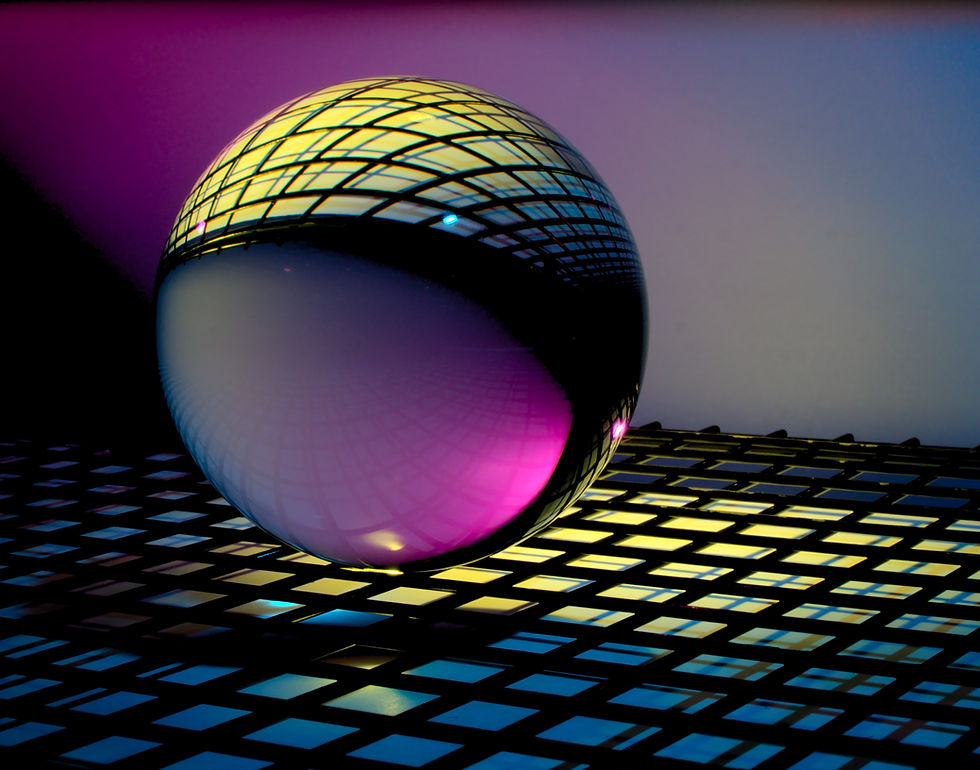
Importance of Germanium in Quantum Computing
The key active component in practically all modern electronics is a transistor, which is why these semiconductor switches are considered to be one of the greatest inventions of the 20th century. Simply put, transistors are tiny on and off switches that represent the standard computer binary system – OFF being a zero state and ON being the one state. Despite silicon having several advantages like a wider band gap resulting in less draining power, better thermal conductivity, and abundance in nature, scientists are resurrecting germanium as a transistor material for quantum computing. In the modern era, the field of quantum technologies has germanium as the emerging and versatile material to make devices that do encoding, process, and transmit quantum information.
Ge has an energy band gap of 0.72ev and Si has an energy band gap of 1.12ev, thus Ge has a higher conductivity, therefore it is considered as a key material to extend chip performance in computers beyond the limits imposed by miniaturization. In the list of semiconductor materials, germanium is not the only high-mobility material. The III-V compounds, such as gallium arsenide and indium arsenide, also possess excellent electron mobility. In fact, the mobility of electrons in indium arsenide is nearly 30 times that of the mobility of electrons in silicon. But there is a problem, this amazing property is not extended to the holes in indium arsenide, which are not much more mobile than holes in silicon. When close to room temperature, the electrons in Germanium move nearly three times as readily as they do in silicon. And the holes-the space that is lacking in an electron-move about four times as easily. The faster these holes and electrons can move, the faster the resulting circuits can be. And since less voltage is required to overcome the band gap and draw those charge carriers along, circuits can also consume considerably less energy. This means the flipping of the switch is much faster in a germanium transistor than a silicon transistor and the Ge current-carrying channel allows moving current at greater rates. Building transistors with such channels could help engineers continue to make faster and more energy-efficient circuits which make germanium a more potent material for operating with qubits, the basic unit of quantum information, which would mean better computers, smartphones, and other gadgets for years to come.
Investment Trend
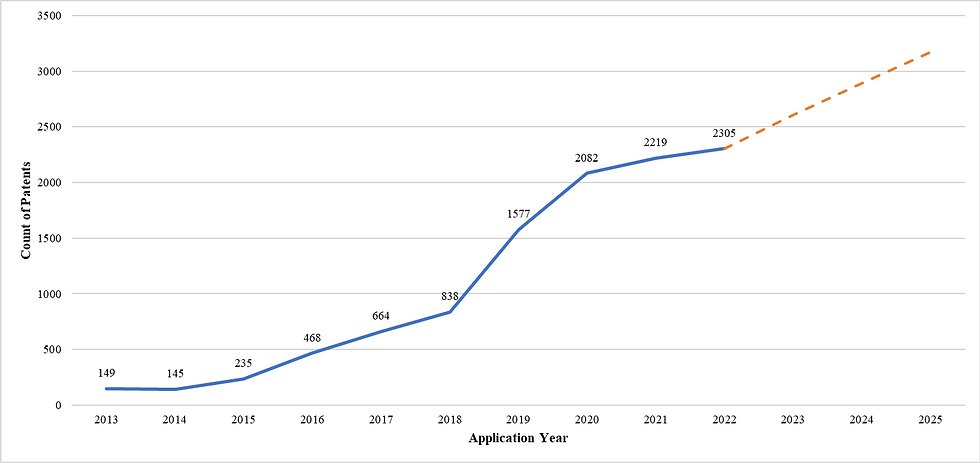
The data shows the number of patent families filed in Quantum Computing technology in the first application year. Quantum computing patents were filed in great amounts from 2015 onwards in the areas of healthcare, environmental systems, energy, fintech, smart materials, and cybersecurity and now the markets have matured in suitable geolocations, so the IT industry is expected to be excited about filing more patents and evolve the capabilities of quantum computing technology. Google also announced Quantum Supremacy in October 2019 with their 54-qubit Sycamore processor chip that performs the computation in 200 seconds. This led to countries and companies rising to the competition and that makes it evident that the quantum race has just started, and it’s not just between major nation-states but also between the industry leaders like Google and IBM.
Top 10 Players
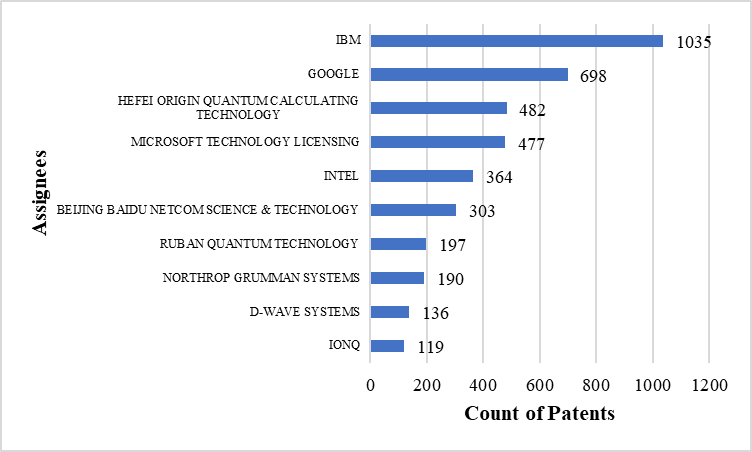
The data shows that IBM filed the most number of patents in the field of quantum computing. IBM has announced that “it is planning to build 1,000-qubit computers from their present 65-qubit computer over the next three years”. Industries like manufacturing, financial services, and security are currently leading the way by experimenting and developing advanced prototypes with more potential use cases and further showing results in their implementation status.
How Does Quantum Computing Work?
Two key breakthroughs are fuelling the renaissance of Ge-based materials and technologies. The first is the maturity achieved by Ge-compatible dielectrics with a high dielectric constant (κ), like aluminum oxide, that overcomes the lack of a stable native oxide; and the second is, the heterogeneous integration of Ge on Si within a conventional complementary metal-oxide-semiconductor process bypasses the need for developing Ge substrates at prohibitive manufacturing costs.
The gate insulator in the state-of-the-art transistor prevents the gate and channel from short-circuiting and plays a critical role. There is no way to create a perfectly flat surface, such that the atoms that sit on the top of the channel will always have a few dangling bonds. So a process called passivation is done to get an insulating layer that links up with as many of those dangling bonds as possible is required. If it isn’t done well, an “electrically bumpy” channel, full of places where charge carriers can get temporarily trapped, lowering mobility and therefore the speed of the device is produced. Silicon has a high-quality “native” insulator that matches up well with its crystal structure: silicon dioxide (SiO2). Germanium has a native oxide that the other III-V materials and Gallium arsenide do not have. In theory, it should mean that Ge has an ideal material to passivate a germanium transistor channel. But there is a problem germanium dioxide (GeO2) is weaker than SiO2, and it absorbs or even gets dissolved by the water used to clean wafers during the chip manufacturing process. Also, it is hard to control the GeO2 growing process which makes it an even worse matter. A layer of GeO2 1 or 2 nm thick is needed for a state-of-the-art device, but it is difficult to make layers thinner than about 20 nm. Therefore, first, a nanometer-thick layer of high dielectric constant insulator, aluminum oxide, is grown on the germanium channel. Once this layer is grown, the ensemble is placed in an oxygen-filled chamber. A fraction of oxygen is passed through the aluminum oxide layer to the underlying germanium, mixing it with the germanium to form a thin layer of oxide (a compound of germanium and oxygen but technically not GeO2). In addition to helping control the growth process, the aluminum oxide acts as a protective cap for this weaker, less stable layer.
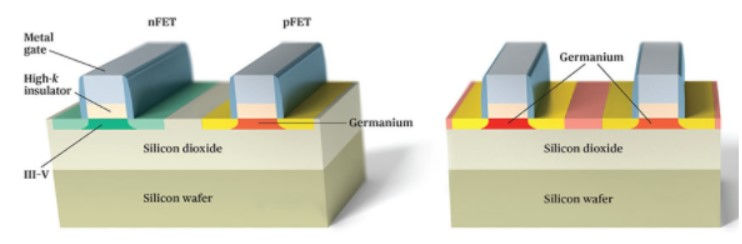
Alternative Channel Paths: There are multiple ways to create transistors with high-mobility, non silicon channels. One approach is to build the nFETs from III-V compounds and the pFETs from germanium, growing patches of both materials on an insulator-topped silicon wafer [left]. As an alternative, both CMOS transistors can be built out of a solid layer of germanium [right], which can be bonded to a silicon wafer (also topped with an insulator).
The high hole mobilities of Ge, benchmark it as an ultra-clean material platform for high-quality and well-controlled quantum dots, which are small nanoparticles having their electronic properties governed by quantum mechanics. Pairs of quantum dots can serve as a single qubit in a quantum logic device. The low effective mass, tunable by confinement and strain, gives quantum dots with large energy level spacing allowing to relax of lithographic fabrication requirements. Uniformity and ease of fabrication are critical in scaling up to large quantum systems. The hyperfine interaction is suppressed due to the p-type character of the valence band of Ge, and it can be engineered by isotopic purification into a nuclear spin-free material leading to long hole-spin lifetimes. Other important properties of holes in Ge that affect the energy levels are the tunable and large g-factors and spin-orbit interaction energies that make Ge desirable for quantum technologies.
From a fabrication perspective, virtually every metal on Ge shows a Fermi level pinned close to the valence band, including superconductors. As a consequence, it is straightforward to make ohmic contact with confined holes in Ge, without the need for local doping or implantation with an associated high thermal budget. Thus, the fabrication of hybrid devices of quantum dot and superconducting structures is majorly due to the resulting strong coupling between metal and semiconductor.
Furthermore, a key building block in semiconductor-superconductor hybrids is the low Schottky barrier at the metal/semiconductor interface that facilitates the formation of transparent contacts to superconductors. Most importantly, Ge is a foundry-compatible material that enables advanced device manufacturing and integration. This is crucial for advancing large-scale quantum systems as many challenges related to epitaxy, dielectrics, and variations of critical device dimensions may be solved by resorting to advanced process control in a state-of-the-art manufacturing facility. The advances in Ge-based materials and the physical understanding of its physical structure and quantum properties have led to impressive achievements and the development of three materials platforms emerging as strong contenders in the race to build quantum information processing devices in germanium: Ge/Si core/shell nanowires (NWs), Ge hut wires (HWs), and Ge/SiGe planar heterostructures. Each of these platforms offers specific advantages to build upon but also poses challenges overcoming to advancing the Ge quantum technology.
Conclusion
We are on the cusp of computer technology that is currently intractable for classical computers. Industry leaders are racing to build advanced Quantum Computing solutions that would provide the computing power required to solve problems that are impossible to do in a timeframe that’s practical with classical computers. With the unique combination of intrinsic materials properties and compatibility with the existing complementary metal-oxide-semiconductor technology, Ge is a promising material within the current second quantum revolution, in which quantum matter is studied to develop technologies beyond the reach of classical understanding.
Comments